From a note in a diary to a state award: Valerii Zapasskii is awarded the Russian Federation National Award
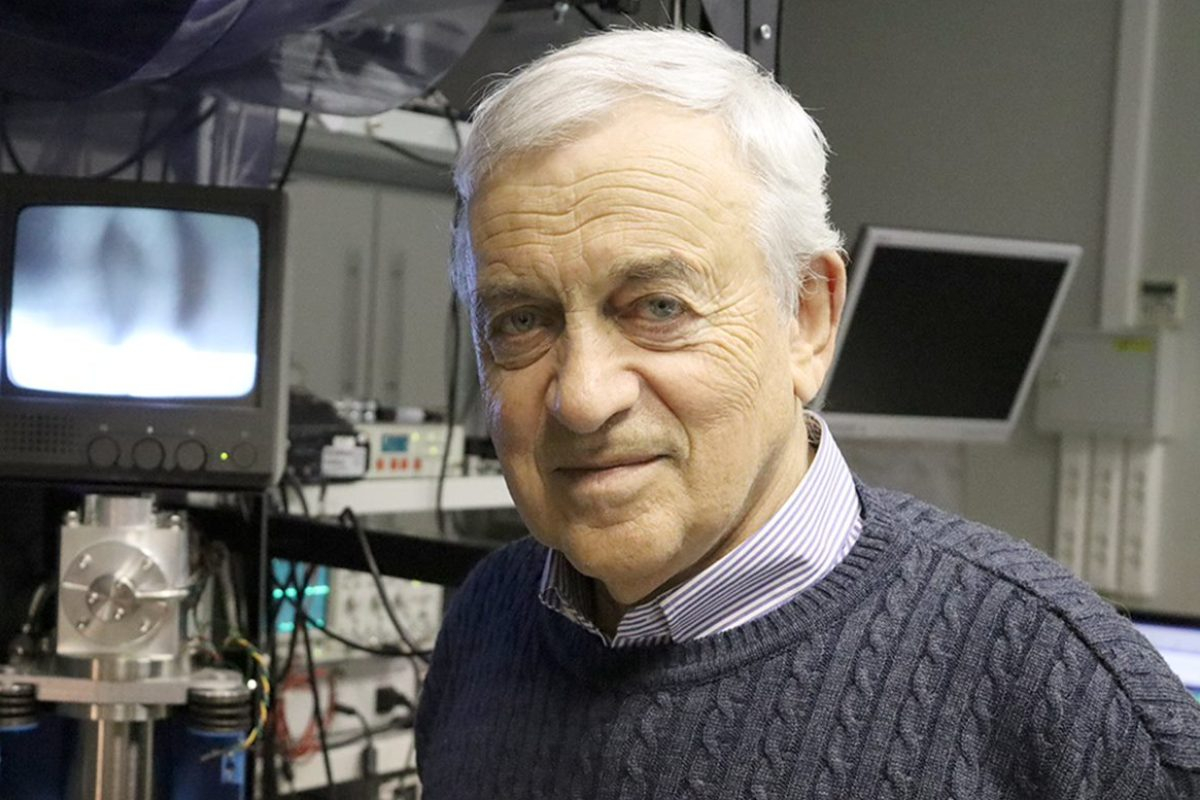
The title of laureate of the 2020 National Award in Science and Technology has been awarded to Valerii Zapasskii, Doctor of Phisics and Mathematics and Leading Research Associate at the Uraltsev Spin Optics Laboratory, St Petersburg University. The annual awards ceremony takes place in the Kremlin.
The Russian Federation National Award is the highest award for scientific and cultural achievements and contribution to society and the state. It is awarded annually in several categories. Russian citizens receive it for discoveries and achievements in science and technology, the results of which have significantly enriched Russian and world science and have had a significant impact on scientific and technological progress.
The research conducted by Valerii Zapasskii has been recognised by scientists worldwide. His work played a key role in the development of a new field in nuclear magnetic resonance – spin noise spectroscopy. In the early 2010s, it was actively developed at the Uraltsev Spin Optics Laboratory at St Petersburg University. This interview with the scientist will give some information about his choice of scientific path, research and achievements.
Dr Zapasskii, how did you get involved in spin research? What exactly made you interested in optics and photonics?
You know, I didn't initially have much interest in optics or photonics. When I was young, my dream was to be a sailor, and then I considered studying astronomy. But my father talked me out of it and I entered the Faculty of Physics. I studied solid state physics at the Faculty of Physics and worked under the supervision of Evgenii Gross. After graduation, I joined the State Optical Institute, where I worked together with my very good friend Vladimir Ovsiankin. That's when optics became a part of my life. We started working in the laboratory of Petr Feofilov, Corresponding Member of the Academy of Sciences of the USSR. Soon after joining the State Optical Institute, Petr Feofilov invited me to take up magneto-optics of impurity crystals.
The method of spin noise spectroscopy has been applied for decades. How did it emerge?
During my first three years at the State Optics Institute, I studied various research methods and became interested in the sensitivity of polarimetric measurements. We discussed this topic with Eugene Alexandrov, Member of the Russian Academy of Sciences and Doctor of Physics and Mathematics. We came to the conclusion that it was possible to increase the sensitivity of polarimeters by 3 to 4 orders of magnitude (i.e. by 1,000 or 10,000 times).
This required developing and building an installation: adapting a magnet, finding a light source (lasers were used very little back then) and photodetectors with high quantum output to name just a few. In short, we made a polarimeter with the maximum achievable sensitivity. All this was new and exciting.
I was impressed by the scale of polarimetric sensitivity in relation to real paramagnetics. If all the spins in our sample are aligned along the field, their rotation inputs will add up, they will rotate the plane of light polarisation by about 50 degrees. If all spins are randomly oriented and have a life of their own, moving and precessing, their total contribution to the rotation will never go down to zero values because of this randomness. This residual quantity should easily be registered with our method, which could register one-hundred-billionth of a full 50-degree rotation, and we would see the ‘wiggling’ of spins and their processional motion. This idea was recorded in my diary in 1975.
When were the first experiments conducted?
We carried out our first experiment on sodium atoms in 1981. I came to Eugene Alexandrov with the idea of spin noise, which back then was called magnetic resonance in the Faraday rotation noise spectrum. At that time, he was working on intensity noise to learn what kind of information they could yield. We immediately found common ground. The experiment was carried out on sodium atomic vapour and the detection was done using a laser polarimeter we developed. We observed the change in the Earth's magnetic field and noticed a peak in the noise at the precession frequency. With an increase in the magnetic field, this peak shifted, i.e. it reflected the magnitude of the magnetic field.
Thus, we developed a new method of registering magnetic resonance. We were very excited, I must say, but we realised that it was of purely academic interest. When we attempted to publish the results abroad, we were told that the benefits of the method were uncertain. So, we submitted the article to the national Journal of Experimental and Theoretical Physics.
This was followed by the study of semiconductor systems and then of dielectric crystals. However, the method was not extensively applied until the turn of the century. In some of the first attempts by foreign researchers to apply the spin noise method to semiconductor crystals, the accumulation time required to observe a signal amounted to about six hours. Understandably, there was little practical value in such a methodology. Since then, the sensitivity of measurements has been increased by several orders of magnitude through the use of special electronic techniques of signal accumulation, and spin noise spectroscopy has advanced considerably.
Please, tell us about the method and the work of your research team.
To answer this question, it is necessary to explain what spin is. Many atoms (paramagnetic atoms) have their own magnetic moments, called spins, which, when a magnetic field is applied, are partially aligned along it and can (like ordinary spinning wheels) precess around the applied field. Spin is a universal thing in nature. Therefore, in any medium containing paramagnetic atoms in a magnetic field there is a motion called precession; all spins seem to ‘buzz’ at the frequency of this precession. Precession is widely used in modern physics. It is this ability of elementary magnetic moments to precess (or ‘buzz’ at a certain frequency) that we focus on.
The problem is how to detect this ‘buzzing’. We do it by using light. The method of spin noise spectroscopy is based on detecting weak fluctuations in the magnetisation of a medium by using polarised light. A linearly polarised laser beam passes through the medium and records information on the spectrum of its magnetisation noise, leaving the medium intact. This information contains interesting data about the spin properties of the medium. In this respect, the spin noise spectroscopy method differs radically from traditional spectroscopy methods where an external stimulus and the response of the medium to it are used to examine the properties of the medium.
These kinds of experiments involve the use of state-of-the-art equipment. What do you use?
The unique equipment for the study of spin noises was developed at the Uraltsev Spin Optics Laboratory. It meets the highest world standards and makes it possible to carry out experiments with maximum measurement sensitivity
The sample, visually a rather unsightly crystal or semiconductor wafer, is placed inside a cryostat and cooled to the temperature of liquid helium (a temperature close to absolute zero). It is exposed to laser radiation with preset parameters for wavelength, intensity and polarisation. The plane of polarisation of the light starts vibrating in the output. It means that its properties are modulated by the chaotic precession of spins of all particles in the medium. Then the beam is directed to the balance detector. In front of the balance detector, the light is divided into two channels, thereby excessive light noises are suppressed and only the polarisation noises remain. With an increase in the applied magnetic field, the frequency of the detected precession signal increases as well.
The signal from the photo detector is transmitted to a fast spectrum analyser, which digitises the analogue data and then constructs a spectrum. This spectrum shows the magnetic resonance.
We have described this process in detail in our publications (e.g Zapasskii, Valerii S. Spinnoise spectroscopy: From proof of principle to applications // Advances in Optics and Photonics. 2013. Vol. 5, Issue 2. P. 131–168). More information about it can also be found on the laboratory’s website.
Dr Zapasskii, could you explain the significance of the spin noise spectroscopy method?
Spin noise spectroscopy is a method of physical research which allows one to observe the motion of the elementary magnets of a medium: atoms, electrons, atomic nuclei and others. An important feature of this method is that it does not measure the response of the medium to external stimuli. Normally, to determine the elasticity of a medium, it is necessary to try to deform it, and to learn the musical tone of a string, it is necessary to stimulate its vibrations. With this analogy, spin noise spectroscopy implies that it is possible to hear the sound of a string when it is resting.
What difference has the establishment of the Uraltsev Spin Optics Laboratory made to the environment for the experiments?
A radical new technique and approach emerged: from scanning spectrum analysers to Fourier analysers. This should have been obvious, but it was not immediately apparent. As a result, it was possible to demonstrate the application of the spin noise technique to semiconductor systems in Fourier spectroscopy in real time.
An important step in the development of this field at St Petersburg University was the award of a mega-grant from the Russian government in 2011 which helped Alexey Kavokin (Another step towards a quantum computer: physicists unveil the condensation of ‘liquid light’ in a semiconductor crystal just one-atom-thick), then a foreign scientist, to set up a new modern experimental facility, the Uraltsev Spin Optics Laboratory. At this stage, we purchased the equipment to build a very advanced spin noise spectrometer and set up a team to work on this project.
During the last decade, spin noise spectroscopy has produced a number of groundbreaking results in the optics of semiconductors, atomic and dielectric systems. What are the future prospects for this method?
Valerii Zapasskii, Doctor of Physics and Mathematics, is a graduate of the Faculty of Physics at Leningrad State University and a distinguished scientist recognised by the international scientific community. Since 2005 Dr Zapasskii has been working at St Petersburg University, first as a staff member of the Department of Photonics, and since 2011 as a leading research associate at the Uraltsev Spin Optics Laboratory, established as a result of a grant from the Government of the Russian Federation. Together with Eugene Alexandrov, Member of the Russian Academy of Sciences and Doctor of Physics and Mathematics, Valerii Zapasskii developed spin noise spectroscopy, a new scientific method of physical research.
Spin noise spectroscopy has revealed a whole new range of possibilities and unique properties. It is now widely used in science and technology to study the properties of semiconductor structures and the energy structure and dynamics of spin systems. This is a unique method that uses noise instead of regular signals.
It is currently being developed to accumulate further knowledge. More recently, we have learned to apply it to impurity dielectric crystals, which previously seemed impossible. These are crystals that are used in laser technology as active media. Now they are also used in quantum memory devices.
Many modern applications use crystals with an admixture of paramagnetic ions. The first analyses demonstrated that it is impossible to apply noise spectroscopy due to the sensitivity. However, we eventually developed an approach based on properties of formation of optical transitions of these systems, and it allowed us to increase the sensitivity of measurements by many orders. What is necessary is a laser with a very narrow bandwidth. This is unique equipment with a whole series of requirements.
One of our more recent advances is the study of anisotropic crystals. Most crystals are anisotropic, which means that they have different optical properties in different dimensions. The light that goes through such crystal has a distorted polarisation, but not because it has precessed spin, but because the crystal is structurally ‘bad’. Now we have received the first results on anisotropic crystals, which is great progress as in the past it was considered impossible.
Then we demonstrated that spin noise spectroscopy can be applied not only to the study of electron spin dynamics, but also to nuclear spin dynamics. This is a whole different story. The nuclei of atoms in a solid body also have spin, they can be oriented, and they precess too. However, if you orient them in a particular way (we do it with light), they relax, and in the process of relaxation the electrons which show the dynamics of the nuclei will be in a different magnetic field. As the nuclei relax, the magnetic field they create also relaxes, and the frequency of precession of electrons changes. By monitoring this frequency, we monitor the nuclei. This is also very interesting.
As you can see, the method continues to surprise us constantly with new discoveries, and much of its fundamental potential remains unexplored.
Dr Zapasskii, can you tell us how you generate new ideas for research and experiments?
Ideas arise in some amazing ways. It is not clear how they emerge. Sometimes you just check the observations you have against some delusional assumptions. Of course, things usually turn out to be wrong. However, in the process of this search, moving through all sorts of hypotheses, you sometimes come across some plausible real effects.
I have always liked observing phenomena. People often work with objects: they take a crystal and examine it from all sides, but I have wanted to observe effects and phenomena all my life. Eugene Alexandrov always talks about the research strategy: if you find a new effect, the first thing to do is to make every effort to suppress or eliminate it. But if it cannot be suppressed, then it must be looked into. I always follow this principle too; it determines the quality of the research. Otherwise, you might announce a discovery and then repent it.
Scientists all over the world are showing interest in the method of spin noise spectroscopy. What would you consider the first thing that attracts them?
This method is an excellent tool. When it came out, scientists were certainly interested in the purposes that it was suitable for.
Speaking generally, spin is now considered very promising for future applications: quantum magnetometry devices, quantum memory devices, and quantum telecommunications. Spin systems are already in use, and this technology continues to develop. Scientists are seeking new strategies for controlling spin in various nanostructures and solid-state materials. This could open up new perspectives in the development of the IT industry. As a research tool, spin noise spectroscopy is of great use.
As another example, it is possible to mention that tomography can be based on this method. When an object is scanned with conventional optics, the light is absorbed and refracted, depending on the path of the light. You cannot select one point on the path of the light and remove the rest: they mutually affect each other. But spin noise spectroscopy allows you, if you focus the beam, to create a point in the medium that you can wiggle around and study its inhomogeneity. It's a completely unconventional and more mobile method than conventional CT and MR-imaging.
There are about a dozen laboratories in the world that study spin noise spectroscopy – in America, Japan, France, Germany. We cooperate with them. Moreover, the Uraltsev Spin Optics Laboratory has a leading place among these laboratories.
What is your advice to aspiring scientists who are choosing their path in science?
I would probably advise them to focus on the creativity of scientific work and enjoy it. This is the best indicator of the success of the work, and incidentally, of living a happy life.
Valerii Zapasskii's research team at the Uraltsev Spin Optics Laboratory at St Petersburg University includes Gleb Kozlov and Ivan Ryzhov, who has been part of the team since his master’s degree and has become a Candidate of Physics and Mathematics and received a presidential grant in 2018 to research spin noise spectroscopy (No MK-2070.2018.2 'Fluctuation Magnetometry'). In 2018, Mikhail Petrov, Senior Research Associate in the laboratory, joined the team and received a grant of the Russian Science Foundation to study spin noise in atoms. Head of the laboratory Alexey Kavokin and Corresponding Member of the Russian Academy of Sciences Mikhail Glazov from the Ioffe PhysicalTechnical Institute are actively involved in the experimental and theoretical tasks of the team along with undergraduate and postgraduate students. Important scientific results were obtained in cooperation with the Manfred Bayer Laboratory of the Technical University of Dortmund, with which the St Petersburg University is implementing a 12-year project funded by the Russian Science Foundation and the German Research Foundation (DFG). In 2020, this project became the winner of the Russian-German competition ‘Russia and Germany: Scientific and Educational Bridges’ (Three St Petersburg University projects are named winners of the international competition ‘Russia and Germany: Scientific and Educational Bridges). The research group is now implementing the project ‘Fundamental Problems of Spin Noise Signal Formation’ run by the Russian Foundation for Basic Research and the German Research Foundation.